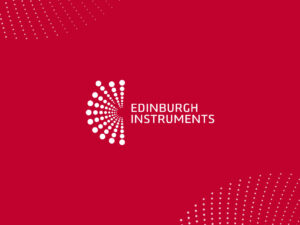
Multichannel Scaling (MCS) is a photon counting method for measuring phosphorescence lifetimes. Multiple photons are collected in each sweep of the time window to quickly accumulate decays across times scales from several hundred nanoseconds to seconds.
In an MCS measurement a pulsed excitation source such as a laser or flashlamp is used to excite the sample and the resulting photoluminescence decay is recorded. The operation of an MCS measurement is shown in Figure 1 and is based on a time sweep that sorts the detected photons into different arrival time channels to create a histogram of the photoluminescence decay. The system comprises of a detector for the emitted photons, which passes the voltage to the constant fraction discriminator (CFD) which produces an electronic signal independent of amplitude. These electronic signals are sorted into bins within the memory (MEM) to form the histogram of time against intensity.
Figure 1. Simplified overview of MCS with two inputs (SWEEP START and Sample SIGNAL) producing a histogram of timed events binned into channels split evenly across the time range.1
The measurement time window is split into channels as shown in Figure 2. A time sweep is started, and the excitation pulse excites the sample. The sweep starts at channel 1 and any photons that arrive during channel 1 are recorded in the memory, the sweep moves onto channel 2 and the photons that arrive during channel 2 are recorded and so on until the sweep has covered the entire window. In Figure 2, two time sweeps are shown, and 11 photons are recorded.
Figure 2. An example of MCS. The time window (orange) is swept by collecting counts within a set number of channels (blue) multiple times to build up the decay. Photons 2 and 3 arrive within the same channel and therefore appear as arriving at the same time.
When multiple photons arrive within the same channel they are recorded as having the same arrival time. The time resolution of the MCS measurement is determined by the time width of the channels in the sweep. Time resolution can be increased by adding more channels, which is typically limited to a maximum time resolution due to constraints on the electronics. This lack of temporal resolution makes MCS unsuitable for photoluminescent decays with lifetimes under 100 ns such as fluorescent emitters, but it is ideal for phosphorescent emitters.
An example of a single exponential phosphorescent decay acquired using MCS is shown in Figure 3. The data has been tail fitted from the peak of the decay to the baseline to give a single 119 µs lifetime component.
Figure 3. Phosphorescence decay of a Europium complex (blue), the single exponential tail fit (red), and the residuals of this fit (grey). Measured on an FS5 Spectrofluorometer using a Xenon microsecond flashlamp for excitation.
Samples may exhibit short, fluorescent decays and longer phosphorescent decays. Both cannot be recorded in high resolution simultaneously, but MCS may be used to isolate the phosphorescent components by using time gating. Time gating removes the fluorescent components from the histogram by switching off the detector at earlier arrival times, effectively blinding the detector to these photons.
An alternative approach to recording photoluminescence decays is the more common time-correlated single photon counting (TCSPC) technique. The key difference between TCSPC and MCS is that in TCSPC only a maximum of one emitted photon can be detected per excitation pulse, while MCS can detect multiple photons per excitation pulse.
In TCSPC the emission count rate must stay below 5% of the laser repetition rate to reduce the probability of multiple emitted photons arriving at the detector and being missed. If higher count rates are used, pulse pile-up occurs, which causes the recorded decay to be skewed to a shorter decay time.1–3 This is shown in Figure 4, where only two STOP signals are recorded for 6 excitations of the sample.
Figure 4. TCSPC method where a pulsed laser starts the counting, and a single detected photon acts as the stop signal.
When measuring short fluorescence lifetimes TCSPC acquisition times are quick as high repetition rate MHz lasers can be used to record millions of photons of the decay within seconds despite this 5% limit. However, it becomes increasingly slow for measuring longer lifetimes as the laser repetition rate must be decreased. Phosphorescence emitters with lifetimes in the hundreds of microseconds to milliseconds would take hours or even days to acquire using TCSPC.
Because MCS can record multiple photons per excitation cycle, the acquisition time is much lower than in TCSPC, especially when measuring long lifetime decays. The downside of MCS is that it has reduced temporal resolution compared to TCSPC. MCS is therefore the optimum technique for measuring longer-lifetime phosphorescent samples where the femtosecond time resolution of TCSPC is not required, and the higher acquisition speed of MCS is a clear advantage.
Table 1. Comparison of TCSPC and MCS.
Typical Performance | PR2 | SC-41 |
---|---|---|
Repeatability (96 well) | <0.5% | <1.5% |
Repeatability (384 well) | <5% | <3% |
Crosstalk (96 well) | <0.001% | <0.1% |
Crosstalk (384 well) | <0.05% | <5% |
Well-to-well repeatability | <5% | <3% |
Sensitivity | 1 pM fluorescein | 40 pM fluorescein |
Spectral range | 250 nm – 900 nm or 400 nm – 2,000 nm (fibre dependent) | Excitation 250 nm – 900 nm Emission 250 nm – 1,650 nm |
Dimensions (mm) | 401 x 320 x 135 | 320 x 245 x 265 (internal to FS5) |
1 W. (Wolfgang) Becker, Advanced time-correlated single photon counting techniques, Springer, 2005.
2 W. Becker, The bh TCSPC Handbook, 8th edn., 2019.
3 J. R. Lakowicz, Principles of Fluorescence Spectroscopy Third Edition, 2010.